Natalia Casey, a Specialist Biomedical Scientist in Microbiology, looks at gut flora and Alzheimer’s disease.
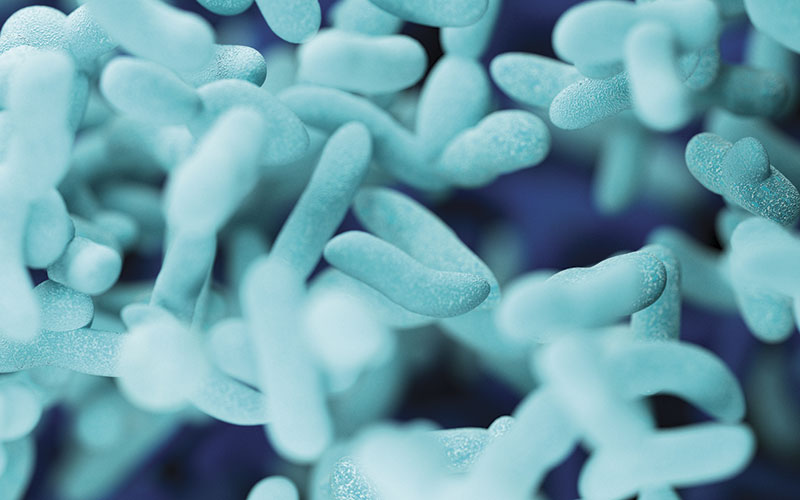
In 2015, it was reported that 48.6 million people were living with dementia worldwide and this is set to increase to 75.6 million by 2030. Definitive diagnosis can only be made at post-mortem, so clinical diagnosis is reliant on psychological and radiological assessments. Because of this, research has focussed on the potential uses of serum and CSF biomarkers, such as protein tau and β-amyloid, and the role the laboratory can play in diagnosing dementias, such as Alzheimer’s disease (AD). In AD, deposition of β-amyloid protein, known as plaques and neurofibrillary tau protein tangles, are characteristic brain developments, resulting in decreased cognitive function and brain shrinkage by loss of neurons and synapses.
Psychologist William James and physiologist Carl Lange introduced the concept of bidirectional communication and emotional regulation between the central nervous system and intestinal organs in the 1880s (Giau et al., 2018). Forty years later, the involvement of the gut microbiota on brain function emerged and the concept of the gut-brain microbiota axis was established.
One of the recent expansions in AD research is the role of gut flora in regulating brain function and modulating neurotransmitters, which can induce β-amyloid deposition and misfolding of tau proteins (tangling) via excessive immune responses. Similar links between the gut microbiota and brain protein function have been implicated in other autoimmune conditions such as multiple sclerosis, and Parkinson’s disease. These conditions are also risk factors for the development of dementias.
Gut microbiota
“There are over 100 trillion micro-organisms in the gastrointestinal tract and a rich diversity of microbes promotes a robust flora against environmental influences”
There are over 100 trillion micro-organisms in the gastrointestinal tract and a rich diversity of microbes promotes a robust flora against environmental influences. In an AD patient, reductions in diversity by decreased numbers of anaerobic bacteria, such as Bifidobacterium, Allobacillum, Akkermansia and Firmicutes,(Vogt et al., 2017) are seen, but data is controversial regarding increases in Bacteroides, which thrive on a meat-rich diet (Mancuso and Santangelo, 2018). Akkermansia reductions are also associated with type 2 diabetes, obesity and increasing β-amyloid protein in the brain (Harach et al., 2017).
The microbial diversity can be reduced by external factors, such as antibiotic induced changes, poor diet and medications such as metformin and proton-pump inhibitors. Central nervous system stress can also negatively affect gut flora and change microbial composition. This is via the hypothalamic–pituitary–adrenal axis which is responsive to these environmental factors and releases the stress-hormone cortisol which, when in excess, can increase both gut permeability and damage the blood-brain barrier. The microbiota can interact with the nervous systems by driving immune response, communicating with the vagus nerve and modulating neuroactive compounds (Strandwitz, 2018).
In terms of neurotransmitter control, organisms such as Escherichia, Bacillus and Streptococcus species can produce dopamine used in motor control and reward-motivated behaviour. Lactobacillus, Lactococcus and Enterococcus species release acetylcholine and histamine, which aid sleep, cognitive function and further neurotransmitter regulation (Giau et al., 2018). An imbalance of these organisms can lead to reduced dopamine and acetylcholine production, leading to cognitive decline via neuronal damage (Francis, 2005). On the other hand, an increased abundance of gram-negative bacteria can produce excessive endotoxin inducing general inflammatory responses and damaging gut and blood-brain barriers.
Excessive immune activation impairs both the gut and blood-brain barrier (Giau et al., 2018), which, in turn, induces neuroinflammation, causes neural injury and ultimately leads to neurodegeneration; a phenomenon that has been termed “inflammaging” (Kowalski and Mulak, 2019). An indirect measurement of gut inflammation is the calprotectin stool concentration. This calcium-binding protein can form amyloid oligomers and fibrils closely resembling β-amyloid and another amyloid-associated protein in AD, α-syn. Calprotectin levels in the CSF of AD patients is significantly increased promoting amyloid aggregation, but this is also seen for stool concentrations in 70% of AD patients (Leblhuber et al., 2015).
Protein alterations
The gut microbiota has been implicated in providing a source of β-amyloid. The most studied bacterial amyloid is curli produced by E. coli. These proteins usually help bacterial cells bind to enable biofilm formation and resist immune responses. Similarities between these amyloids and those found endogenously in the CSF (β-amyloid) have been found, indicating that high concentrations in the gut may strengthen immune responses to amyloid in the central nervous system. Studies in rats have shown that brains exposed to higher amounts of curli-producing bacteria have increased microgliosis, astrogliosis and increased expression of TLR2, IL6 and TNF which can further the inflammatory features of AD. (Pistollato et al., 2016).
Bacteria and fungi in the gut can excrete lipopolysaccharides (LPS), which modify gut homeostasis, inflammation and permeability. Predominantly, these arise from abundant gram-negative bacilli and make-up part of a mixture of pro-inflammatory neurotoxins, that when leaked into the circulatory system and cerebral vasculature, have been found to eventually reside in the neocortex and hippocampus, contributing to neurodegeneration (Zhao et al., 2017). Animal model studies show that LPS injections direct into the brain can result in pathological features, from prolonged elevation of amyloid in the hippocampus, similar to those seen in AD.
Helicobacter pylori associations
Since its first identification in 1982, H. pylori gastrointestinal colonisation has been linked to many conditions, including atherosclerosis, hypertension, stroke and peptic ulcer diseases (Doulberis et al., 2017). The vascular disorders are risk factors for AD, implicated by impairing of the blood-brain barrier via inflammatory responses. A Th1 immune response to H. pylori infection is characterised by high levels of TNF-α, IFN-γ, IL-2 and IL-12 causing gastric epithelial cell apoptotic damage. As well as the cross-reaction of TNF-α inducing neuronal cell death, TNF-α may also be able to contribute to obstructive sleep apnea, which accelerates amyloid deposition in the brain (Doulberis et al., 2017). In addition, intraperitoneal injection of H. pylori in animal models induces memory deficit and increases β-amyloid in the hippocampus and cortex by interrupting synaptic function, just as it can induce tau hyper-phosphorylation creating neurofibrillary tangles (Doulberis et al., 2017).
Microbiota modulation
Dietary intervention is one of the most effective methods of modifying the gut microbiota. Diets rich with plant-based foods, nuts and antioxidants and low in saturated fats and refined sugars have been shown to inhibit inflammatory responses, reduce insulin resistance and decrease the risk of neurocognitive impairment including AD (Kolwalski and Mulak, 2019). This is due to a shift away from Bacteroides to increased levels of other anaerobes, including Akkermansia and Prevotella. Probiotics provide additional support to the microbiota as indigestible substrates act as food for Bifodobacterium and Lactobacilli, whereas red wine, red berries and green tea contribute to reducing amyloid aggregation and the incidence of amyloid-related diseases (Rigacci et al., 2015). Conversely, diets low in antioxidants and high in pro-inflammatory fatty acids facilitate the deposition of amyloid and activate inflammatory processes.
Faecal transplantation has been used since the 4th century for the treatment of diarrhoea and food poisoning. Nowadays, the NHS provides faecal transplantation services to patients with recurrent C. difficile infection with the aim of recolonising the gut. Faecal transplantation has also shown therapeutic potential in neurodegenerative disorders such as Parkinson’s disease, which could be expanded to AD to restore gut microbiota diversity and stability (Evrensel and Ceylan, 2016). It is thought that this could reduce intestinal permeability and decrease immune activation (Bostanciklioğlu, 2018). Although studies are lacking in this area, modulation of gut bacteria could have far-reaching effects to prevent and delay progression of AD. Donor screening programmes are similar to those of blood donors, with a range of bacteria, parasites and viruses tested and the exclusion of donors with gastrointestinal disease history and use of major immunosuppressives.
The lab role
Due to manufacturing requirements, donor samples are unlikely to be seen in the clinical microbiology laboratory. However, we may see requests for proof of recolonisation or gut microbiome determination as a tool for patient management. For example, in a western diet high in protein and fat in the UK, Bacteroides can make up 55% of the microbiome and in North America, this can reach 80%. On a plant-based diet, percentages are tipped towards the Firmicutes, which aid digestion of plant carbohydrates and modulate gut inflammation. Implementation of techniques such as RNA gene sequencing will make microbial ecology an exciting prospect for clinical microbiology and for the management of Alzheimer’s affected patients.
Natalia Casey is a Specialist Biomedical Scientist in Microbiology, at Weston General Hospital. She is studying for an MSc in Global Health.
Image credit | Science Photo Library
References
Akbari, E., Asemi, Z., Daneshvar Kakhaki, R., Bahmani, F., Kouchaki, E., Tamtaji, O.R., Hamidi, G.A. and Salami, M. (2016). Effect of probiotic supplementation on cognitive function and metabolic status in Alzheimer's disease: a randomized, double-blind and controlled trial. Frontiers in aging neuroscience, 8, p.256.
Bostanciklioğlu, M. (2018). Intestinal Bacterial Flora and Alzheimer’s Disease. Neurophysiology, 50(2), pp.140-148.
Doulberis, M., Kotronis, G., Thomann, R., Polyzos, S., Boziki, M., Gialamprinou, D., Deretzi, G., Katsinelos, P. and Kountouras, J. (2017). Review: Impact of Helicobacter pylori on Alzheimer's disease: What do we know so far? Helicobacter, 23(1), p.e12454.
Evrensel, A. and Ceylan, M. (2016). Fecal Microbiota Transplantation and Its Usage in Neuropsychiatric Disorders. Clinical Psychopharmacology and Neuroscience, 14(3), pp.231-237.
Francis, P. (2005). The Interplay of Neurotransmitters in Alzheimer's Disease. CNS Spectrums, 10(S18), pp.6-9.
Giau, V., Wu, S., Jamerlan, A., An, S., Kim, S. and Hulme, J. (2018). Gut Microbiota and Their Neuroinflammatory Implications in Alzheimer’s Disease. Nutrients, 10(11), p.1765.
Harach, T., Marungruang, N., Duthilleul, N., Cheatham, V., Mc Coy, K.D., Frisoni, G., Neher, J.J., Fåk, F., Jucker, M., Lasser, T. and Bolmont, T. (2017). Reduction of Abeta amyloid pathology in APPPS1 transgenic mice in the absence of gut microbiota. Scientific reports, 7, p.41802.
Kowalski, K. and Mulak, A. (2019). Brain-Gut-Microbiota Axis in Alzheimer’s Disease. Journal of neurogastroenterology and motility, 25(1), p.48.
Leblhuber, F., Geisler, S., Steiner, K., Fuchs, D. and Schütz, B. (2015). Elevated fecal calprotectin in patients with Alzheimer’s dementia indicates leaky gut. Journal of Neural Transmission, 122(9), pp.1319-1322.
Mancuso, C. and Santangelo, R. (2018). Alzheimer’s disease and gut microbiota modifications: The long way between preclinical studies and clinical evidence. Pharmacological Research, 129, pp.329-336.
Pistollato, F., Sumalla Cano, S., Elio, I., Masias Vergara, M., Giampieri, F. and Battino, M. (2016). Role of gut microbiota and nutrients in amyloid formation and pathogenesis of Alzheimer disease. Nutrition reviews, 74(10), pp.624-634.
Queensland Brain Institute. (2019). What causes dementia? [online] Available at: https://qbi.uq.edu.au/dementia/dementia-causes-and-treatment [Accessed 5 May 2019].
Rigacci, S. and Stefani, M. (2015). Nutraceuticals and amyloid neurodegenerative diseases: a focus on natural phenols. Expert review of neurotherapeutics, 15(1), pp.41-52.
Strandwitz, P. (2018). Neurotransmitter modulation by the gut microbiota. Brain Research, 1693, pp.128-133.
Valdes, A., Walter, J., Segal, E. and Spector, T. (2018). Role of the gut microbiota in nutrition and health. BMJ, p.k2179.
Vogt, N.M., Kerby, R.L., Dill-McFarland, K.A., Harding, S.J., Merluzzi, A.P., Johnson, S.C., Carlsson, C.M., Asthana, S., Zetterberg, H., Blennow, K. and Bendlin, B.B. (2017). Gut microbiome alterations in Alzheimer’s disease. Scientific reports, 7(1), p.13537.
Zhao, Y., Cong, L., Jaber, V. and Lukiw, W.J. (2017). Microbiome-derived lipopolysaccharide enriched in the perinuclear region of Alzheimer’s disease brain. Frontiers in immunology, 8, p.1064.